Estimated read time: 3-4 minutes
This archived news story is available only for your personal, non-commercial use. Information in the story may be outdated or superseded by additional information. Reading or replaying the story in its archived form does not constitute a republication of the story.
SALT LAKE CITY -- Given the fame and veneration of Albert Einstein as possibly the greatest scientist to have lived, it seems almost impossible that we are still confirming scientific phenomena that he predicted half a century ago. But it's true, and scientists from Australia and Germany are now capable of conducting an experiment that will show that his genius continues to loom over the world of astrophysics.
"By literally squeezing light on a quantum level, we are refining our detection instruments to an extent never seen before," said David Blair, who was part of the team to demonstrate this technique, and who is also the director of the Australian International Gravitational Research Centre at University of Western Australia.
Einsteins theory of general relativity says that gravity ought to come in waves, just like light. The only catch is that the waves are generally mind-bendingly tiny - millions of times smaller than ordinary waves of light.
But certain really fantastic astronomical events, like black holes running into each other, cause gravity waves just big enough to be detected out here on distant planet Earth.
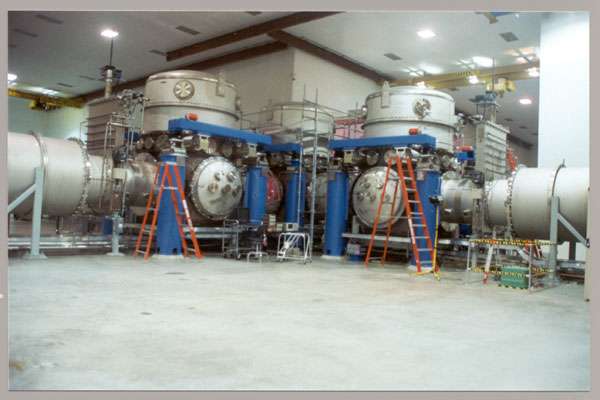
Basically how the experiment works is this: A powerful laser beam is split. The two beams then travel for a while, and are then recombined. Because light has wave- like properties, when the two beams are recombined, any change in the beams as they travel along a path before recombination will cause a disturbance that is measurable when you try to put them back together. It's sort of like if you and friend each went on a walk in opposite directions, and then when you saw each other next, you tried to guess where the other has been based on what's stuck to the other's shoes.
The idea is that if gravity also has wave-like properties, it ought to also cause a disturbance in the lasers' light that is measurable. If you can eliminate any other source of disturbance, you can say with confidence that a gravity wave has been detected.

The problem with the current generation of experiments is that they have so far not been able to eliminate noise in the lasers' signal caused by that pesky Uncertainty Principle which governs the world of the unimaginably small quantum world. As your measurements get smaller and smaller, more and more precise, the world behaves very differently. Particles, all of which have wave-like properties, pop into existence from absolutely nothing, and immediately destroy each other. These are called vacuum fluctuations. If this happens at the site of detection, these particles cause a disturbance in the beams and therefore ruin the results of the experiment. Scientists call this phenomenon "noise."
"Remarkably, we have broken through the quantum measurement barrier that up to now set a limit to the sensitivity of our detectors," Blair said.
Gravity waves are so weak and difficult to detect that even these random disturbances make it impossible to get to the sensitivity required. But that's when scientists decided to use the Uncertainty Principle to their advantage. If you are willing to sacrifice certainty in one area of measurement in order to achieve more certainty in another area, you are basically "squeezing" the light. That is when you plot out the uncertainty in one dimension verses the uncertainty in another; you get an ellipse, or a squeezed circle.
Scientists used the phenomenon of quantum entanglement in order to "squeeze" the light, thus giving them more precise measurements at the site of the detector, where vacuum fluctuations really become a problem.
The technique is novel both for its unprecedented accuracy of measurement, and for its use of quantum entanglement, a phenomenon that itself was only recently confirmed.
Email: [dnewlin@ksl.com](<mailto: dnewlin@ksl.com>)